Tackling quantitative measurement needs at the interface of biology, medicine, engineering, physics, and chemistry
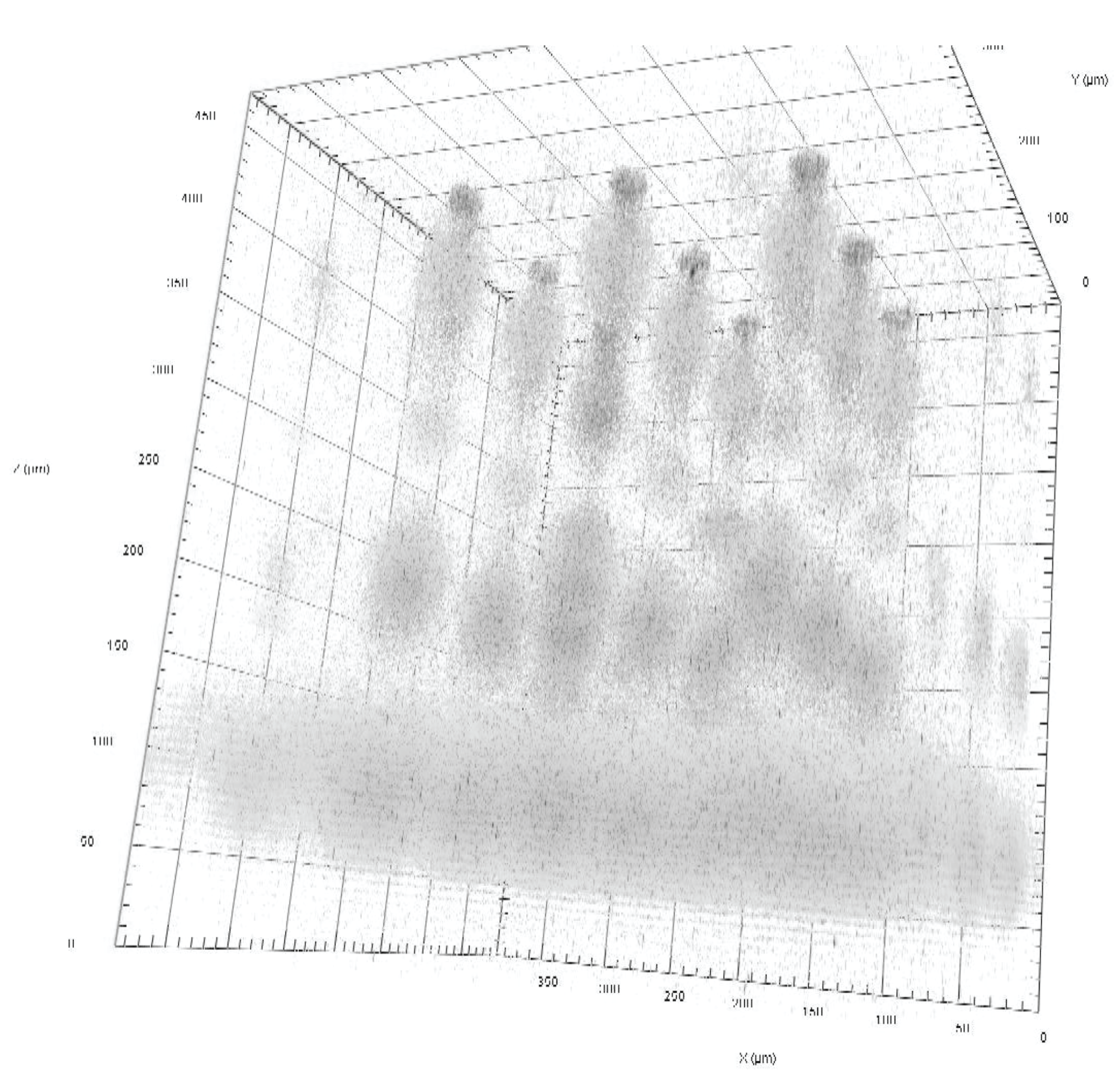
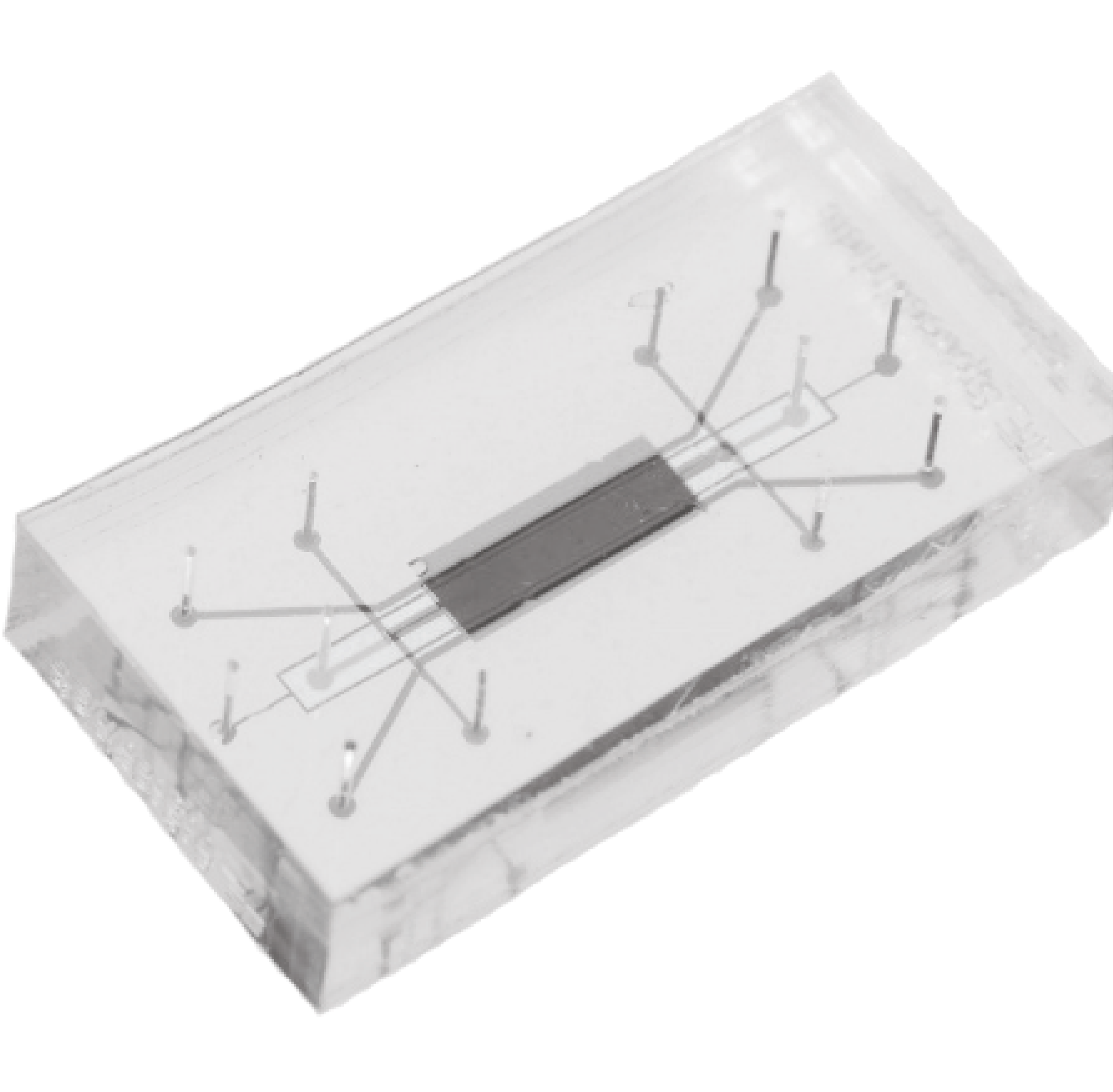
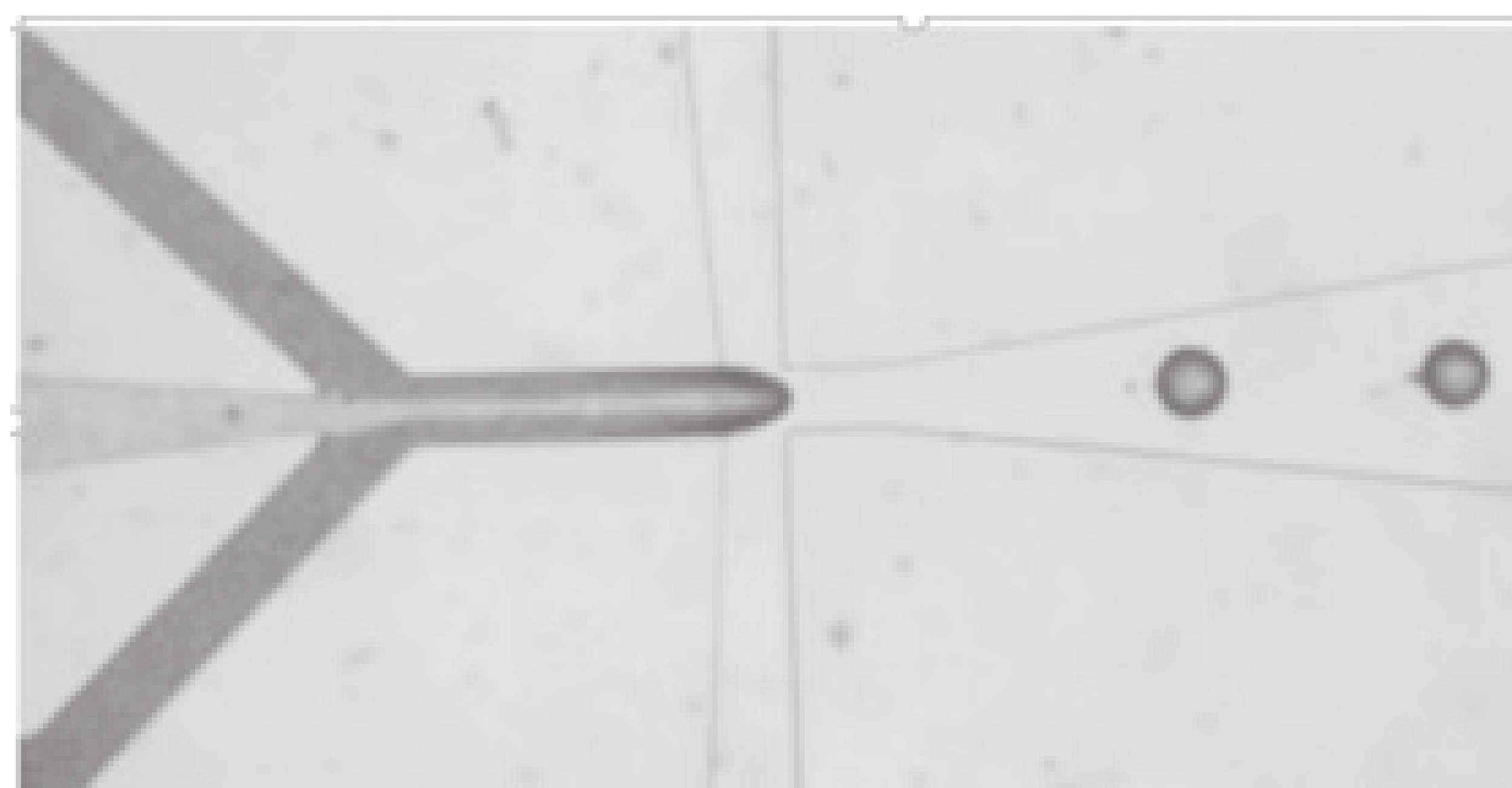
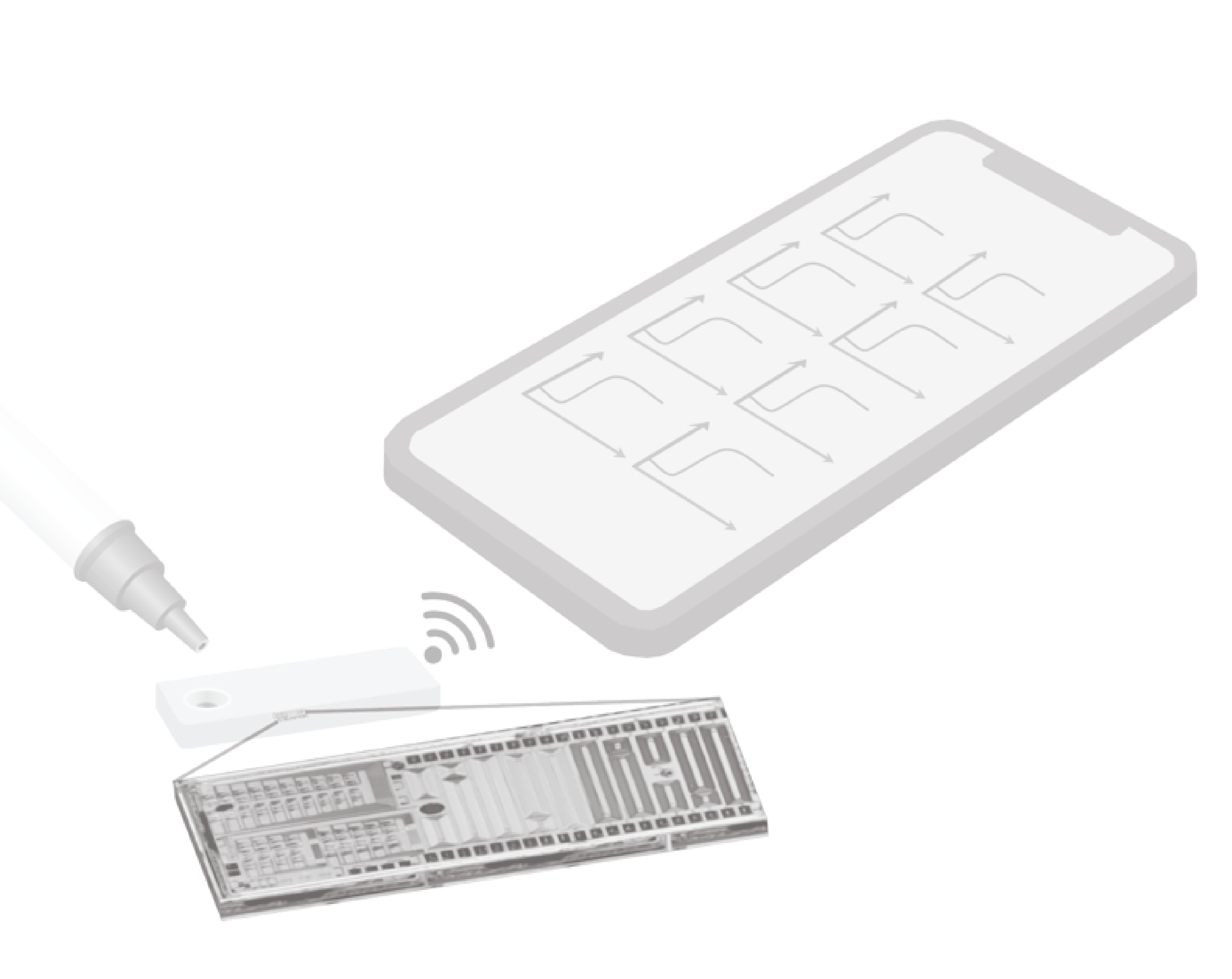
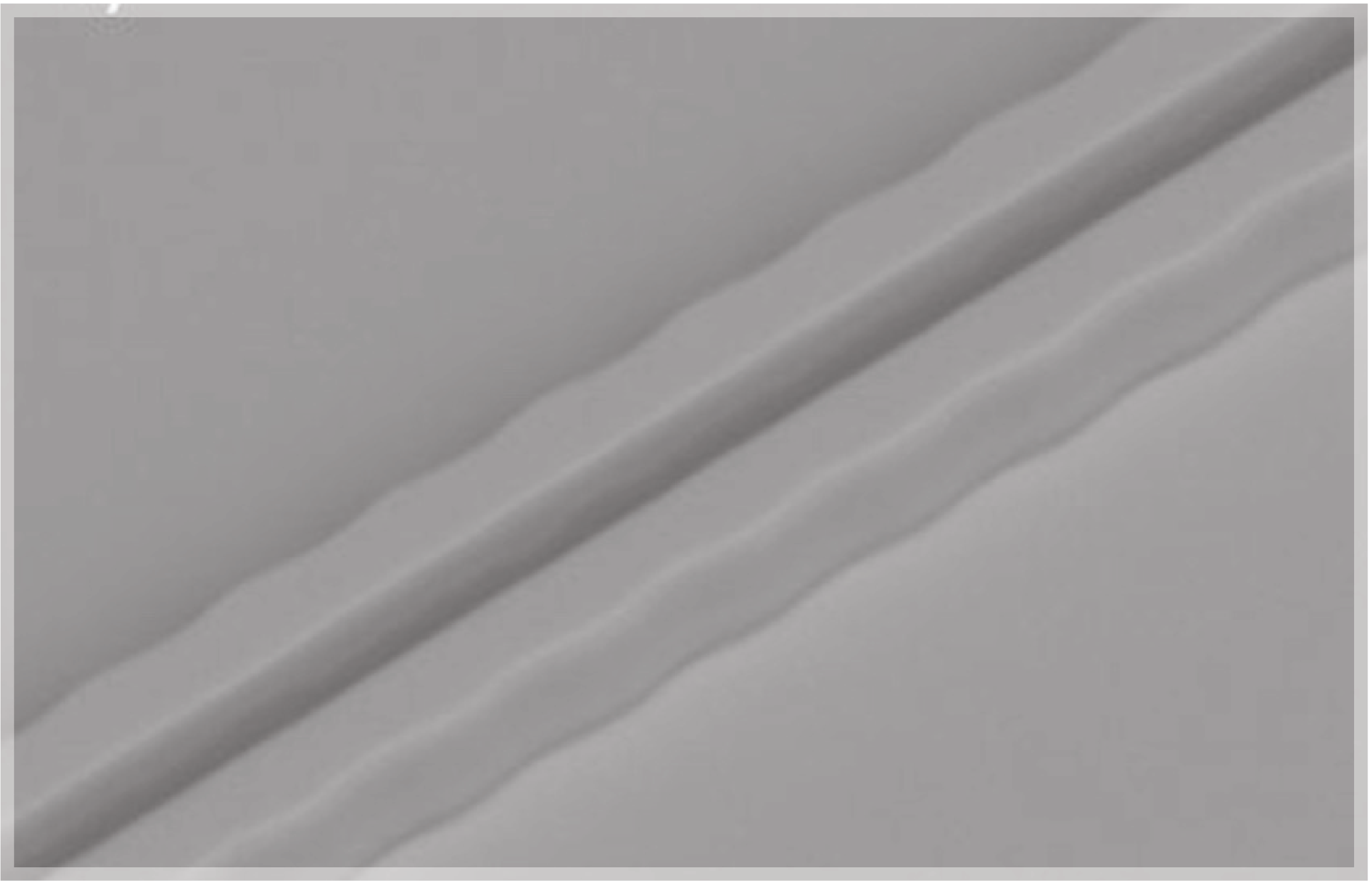
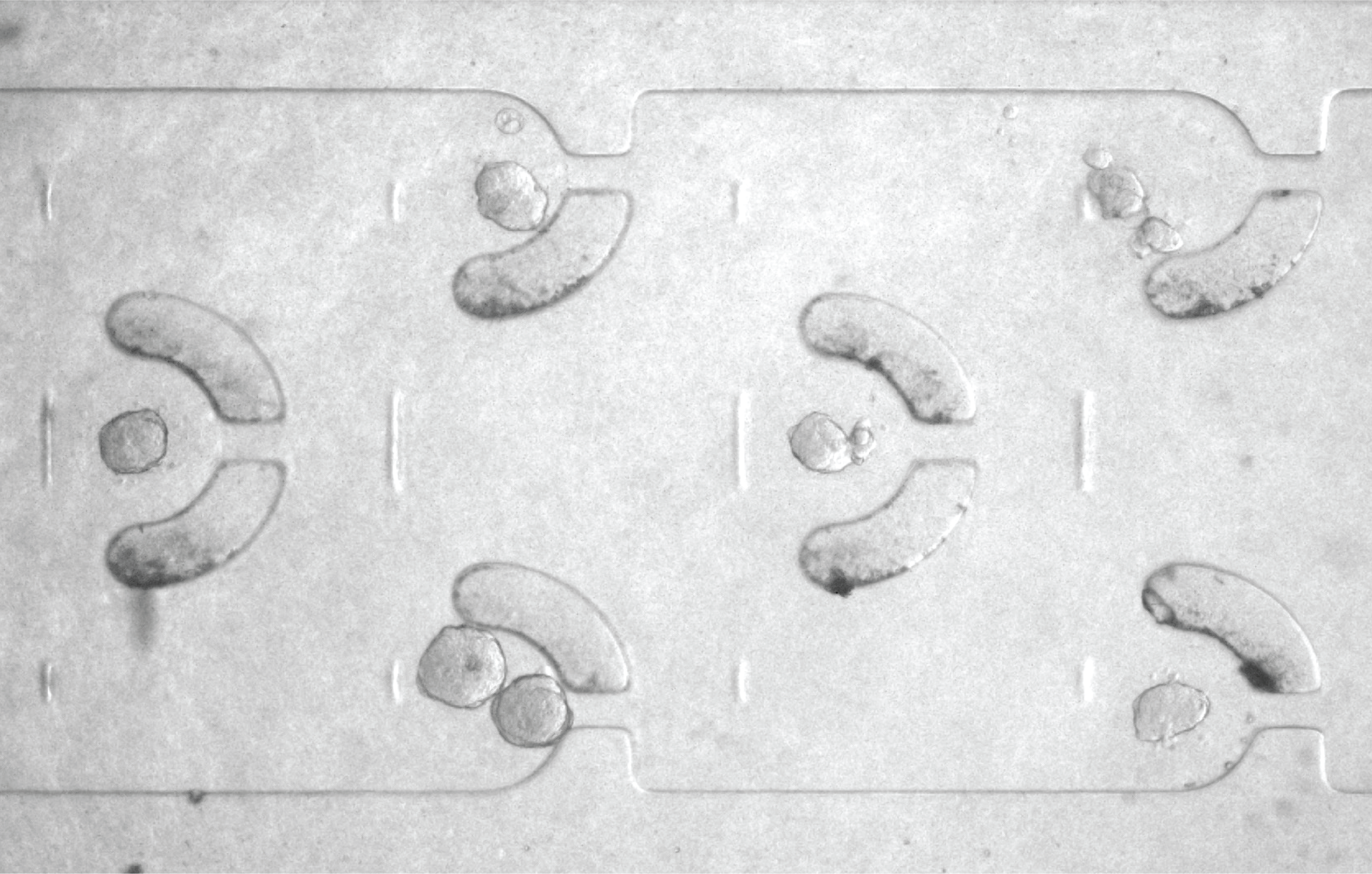
About Samantha
Dr. Samantha M. Grist is a Research Associate in the School of Biomedical Engineering and Department of Electrical and Computer Engineering at the University of British Columbia (UBC). She leads development of silicon photonic biosensors for point-of-need diagnostics applications, including women’s health during the menopausal transition. Dr. Grist’s research interests span several disciplines and focuses on applying principles of engineering science and fundamentals of physics and chemistry to develop tools to better understand biological systems, including:
analytical biosensors to unlock new types of diagnostic measurements.
silicon photonics and electronic-photonic integration miniaturize sensors and systems including biological measurements and biosensors.
robust, quantitative optical sensors to better understand biological and chemical systems, including quantifying critical oxygen levels within biological systems including organs-on-chip, and quantifying ultraviolet (UV-C) radiation during ultraviolet decontamination.
single-cell protein immunoassay measurements to understand heterogeneity in biological systems and links to mechanisms of disease formation and progression.
microfluidic design (including biomimetic devices) to support analytical measurements and recreate microenvironmental conditions in the human body (for example, organs-on-chip to improve in vitro testing of drug candidates).
imaging approaches to reveal new insights about biological systems, including tissue clearing and optical-sectioning microscopy.
At UBC, she works with Professors Karen C. Cheung, Sudip Shekhar, and Lukas Chrostowski. She previously completed an NSERC postdoctoral fellowship in the Bioengineering Department and the California Institute for Quantitative Biosciences at the University of California, Berkeley, working with Professor Amy E. Herr from 2017-2020. She completed her Ph.D. in Electrical and Computer Engineering at the University of British Columbia in 2016, where she was advised by Professors Karen C. Cheung and Lukas Chrostowski, and her B.A.Sc. (Hons.) in Engineering Physics from Simon Fraser University’s School of Engineering Science.
Research
Towards quantitative, portable, data-rich diagnostics using integrated silicon photonics technology
There are unmet needs to bring the quantitative, accurate, and data-rich diagnostics of centralized testing laboratories to the point-of-need — especially for medical applications where frequent testing is required. One important need for this type of measurement is to support health during the menopausal transition, which has been historically neglected. Menopause health suffers from a lack of information about how each woman’s body works and the hormone fluctuations that underlie not only symptoms of perimenopause but also risk for other conditions. Obtaining this information is challenging because it requires frequent (≥2x/wk), non-invasive measurement of several hormones in a convenient setting and cost point. Current technologies are either too expensive or inconvenient for frequent testing (lab/mail-in tests) or cannot measure enough hormones for actionable insight (at-home tests). This urgent gap requires innovation in at-home testing.
To meet these types of needs, we are using a new chip-scale technology to permit accurate, quantitative, simultaneous measurements of multiple hormone or disease markers in a portable, low-cost form factor. Silicon photonics technology integrates optical (light-based) devices and structures into silicon chips like computer chips, routing light on-chip using nanoscale waveguide structures (like tiny optical fibres). Silicon photonics permits miniaturization and integration of 10s-100s of sensors on a mm-scale chip, permitting quantification of ≥10 hormones. Our team has developed a new sensor technology that we anticipate will miniaturize the readout system and support diagnostic measurements at a cost (<$20/test) and form factor suitable for at-home use — while maintaining the same type of data-rich readout. We anticipate that this device will open the door to new types of analytical measurements, empower people during perimenopause, improve the menopause standard of care, and address a key gender disparity in healthcare.
Towards this goal, our team is working on:
quantifying the performance of our new sensor architecture and comparing it to the state-of-the-art.
developing and optimizing sensor functionalization and assay workflows for specific detection.
developing microfluidic devices to deliver sample to the surface of our sensors.
integrating tiny, low-cost lasers into silicon photonics devices for low-cost readout.
optimizing sensitivity and performance of optical waveguides used for optical sensors.
Collaborators:
Professor Karen C. Cheung
Professor Sudip Shekhar
Professor Lukas Chrostowski
Key publications and patents:
S. M. Grist, L. S. Puumala, M. A. Al-Qadasi, A. Randhawa, S. J. Chowdhury, K. Wickremasinghe, S. J. Kim, Y. Oloumi Yazdi, L. G. Alde, Y. Liu, N. C. Tang, M. Mitchell, L. Chrostowski, S. Shekhar, K. C. Cheung. Silicon Photonic Biosensing: Towards Portable Diagnostics at the Point of Need. Presented as an invited conference presentation In Microfluidics, BioMEMS, and Medical Microsystems XXI. SPIE, 2023; p. 7. doi: 10.1117/12.2657809.
S. J. Chowdhury, K. Wickremasinghe, S. M. Grist, H. Zou, M. Mitchell, M. A. Al-Qadasi, B. Lin, D. Birdi, S. Smythe, S. Shekhar, K. C. Cheung, L. Chrostowski. On-chip hybrid integration of swept frequency distributed-feedback laser with silicon photonic circuits using photonic wire bonding. Optics Express 32 (3). 2024. doi: 10.1364/OE.510036.
L. S. Puumala, S. M. Grist, J. M. Morales, J. R. Bickford, L. Chrostowski, S. Shekhar, and K. C. Cheung. Biofunctionalization of Multiplexed Silicon Photonic Biosensors. Biosensors 13 (1). 2023. doi: 10.3390/bios13010053.
L. S. Puumala, S. M. Grist, K. Wickremasinghe, M. A. Al-Qadasi, S. J. Chowdhury, Y. Liu, M. Mitchell, L. Chrostowski, S. Shekhar, and K. C. Cheung. An Optimization Framework for Silicon Photonic Evanescent-Field Biosensors Using Sub-Wavelength Gratings. Biosensors 12 (10). 2022. doi: 10.3390/bios12100840.
L. Chrostowski, S. Shekhar, S. M. Grist, K. C. Cheung, & M. A. Al-Qadasi. US11940386B2 Photonic Sensor Using a Fixed-Wavelength Laser. 2023.
Revealing heterogeneity in cell state with high throughput and high specificity, using a new class of three-dimensional immunoassay
The environment around the cells in our body is crucial to their behaviour — this is one reason pathologists look at tissue-level architecture rather than just individual cells when diagnosing diseases such as cancer. But a major shortcoming of these existing diagnostic technologies is that they lack the specificity required to determine whether the tumour expresses certain biomolecules which can be indicative of cell behaviour or resistance to cancer treatments. At UC Berkeley, I led the development of a new technique with the goal to combine (1) retention of tissue-level architecture with (2) protein specificity beyond that possible with antibodies alone. To do this, we engineered a new tool that separates biomolecules in a 2D pattern of cells by size while retaining their spatial organization. This tool used rapid lysis to release proteins from single cells, electrophoresis to separate the proteins within a 3D hydrogel matrix, smart hydrogels to capture proteins and “freeze” the separation in place, an immunoassay to label captured proteins for detection, and optical sectioning microscopy and data analyses to simultaneously measure protein size and abundance from hundreds of single cells.
Projection electrophoresis simultaneously lyses and separates proteins from hundreds of single cells in parallel, at a throughput of >2.5 cells/s (>70x faster than serial sampling), and requires 10x less sample to analyze the same number of cells as previous immunoblots. Synchronous analysis of cell populations (like that facilitated by projection electrophoresis) is crucial to assessing dynamic cell response to microenvironmental stimuli. This new approach enabled simultaneous single-cell separations (similar to Western blots). Projection electrophoresis also opens the door to measurements preserving tissue architecture. We anticipate that the projection electrophoresis approach will contribute to workflows enabling improved cancer classification and selection of more effective cancer therapy regimens for patients' tumours.
Key publications and patents:
S. M. Grist, A. P. Mourdoukoutas, and A. E. Herr. 3D projection electrophoresis for single-cell immunoblotting. Nature Communications 11, 6237. 2020. doi: 10.1038/s41467-020-19738-1.
A. P. Mourdoukoutas, S. M. Grist, and A. E. Herr. Rapid electrotransfer probing for improved detection sensitivity in in-gel immunoassays. Analytical Methods, 12, 4638-4648. 2020. doi: 10.1039/D0AY01203C.
International patent application WO2019212974A1. Tissue projection electrophoretic separation of protein. Inventors: A. E. Herr, S. M. Grist, C.-C. Kang, B. Gumuscu Sefunc, J. Vlassakis. Published 2019-11-07.
Precision biology: engineering science and microfluidic design to better understand the tumour microenvironment and role of hypoxia in cancer progression
The heterogeneity of cells in our bodies gives rise to well-functioning tissues as well as the dysfunction of disease. Measurements of the cells alone, however, do not tell the full story: heterogeneity is linked to the environment around each cell. The heterogeneous and dynamically-changing microenvironment can influence cell state, impacting fields like cancer, regenerative medicine, neurodegenerative disease, and the microbiome. Despite its importance, the microenvironment is poorly reproduced by most common in vitro testing platforms, and there are also challenges associated with measuring cell state in response to dynamic microenvironmental conditions. In this work, we used engineering science and microfabrication to precisely and spatiotemporally control the cell microenvironment. We developed and applied a microfluidic platform to expose tumour cells in 3D culture to controlled oxygen conditions, recreating the chronic and time-varying hypoxic (low oxygen) profiles characteristic of many solid tumours. These hypoxic conditions have been associated with tumour progression, metastasis, and drug resistance, but they are absent from traditional tissue culture techniques because of constraints from large size scales and material limitations.
We developed a new microfluidic system to provide:
precise control of oxygen levels surrounding 3D cell cultures (down to <0.1% oxygen), with design informed by analytical and computational modelling of convective and diffusive oxygen transport within the microfluidic system.
fast oxygen switching times of <10 minutes to reproduce physiologically relevant profiles of cycling hypoxia that exist in tumours.
capability to create oxygen gradients and spatiotemporal oxygen profiles.
integrated in situ measurement of oxygen levels using integrated optical oxygen sensors to validate oxygen control.
3D cell culture in hydrogel matrices containing important constituents of the natural extra-cellular matrix, with compatibility with advanced microscopy monitoring of cells under test.
controlled delivery of nutrients and drugs to the cells under test.
The microfluidic platform we developed enabled the discovery that breast tumour spheroids swell and shrink in response to cycling oxygen profiles, and also permitted assessment of cancer treatment uptake under controlled oxygen conditions. These types of advances in microenvironment mimicry, combined with cell state metrology enabled by our team’s other research directions including silicon photonic sensors, immunoassay design, and optical-sectioning microscopy, promise to uncover the mechanistic role of the dynamically-changing microenvironment in the diversity of human cell types and cell states, creating a focused impact on prevention, diagnostics, and treatment across several diseases.
Key publications:
S. M. Grist, K. L. Bennewith, and K. C. Cheung. Oxygen Measurement in Microdevices. Annual Review of Analytical Chemistry. 15(1), 221-246. 2022. (Invited Review) doi: 10.1146/annurev-anchem-061020-111458.
S. M. Grist, S. S. Nasseri, L. Laplatine, J. C. Schmok, D. Yao, J. Hua, L. Chrostowski, and K. C. Cheung. Long-term monitoring in a microfluidic system to study tumourspheroid response to chronic and cycling hypoxia. Scientific Reports, 9, 17782. 2019. doi: 10.1038/s41598-019-54001-8.
S. M. Grist, J. Schmok, M.-C. Liu, L. Chrostowski, and K. Cheung. Designing a Microfluidic Device with Integrated Ratiometric Oxygen Sensors for the Long-Term Control and Monitoring of Chronic and Cyclic Hypoxia. Sensors, 15, 20030. 2015. (Invited Feature Paper) doi: 10.3390/s150820030.
S. M. Grist, N. Oyunerdene, J. Flueckiger, J. Kim, P. C. Wong, L. Chrostowskiand K. C. Cheung. Fabrication and laser patterning of polystyrene optical oxygen sensor films for lab-on-a-chip applications. Analyst, 139, 5718-5727. 2014. doi: 10.1039/c4an00765d.
S.M. Grist* and S. S. Nasseri*, T. Poon, C. Roskelley, K. C. Cheung. On-chip clearing of arrays of 3-D cell cultures and micro-tissues. Biomicrofluidics, 10, 044107. 2016. doi: 10.1063/1.4959031.
L. Yu, S. M. Grist, S. S. Nasseri, E. Cheng, Y. C. E. Hwang, C. Ni and K. C. Cheung. Core-shell hydrogel beads with extracellular matrix for tumor spheroid formation. Biomicrofluidics, 9. 2015. doi: 10.1063/1.4918754.
S. M. Grist, L. Chrostowski, and K. C. Cheung. Optical Oxygen Sensors for Applications in Microfluidic Cell Culture. Sensors 2010, 10(7). 2010. (Graduate Work) doi: 10.3390/s101009286.
Awards
2024 UBC School of Biomedical Engineering $20,000 Trainee Research Award research grant: "Towards a quantitative, accurate, portable, and multiplexed diagnostic system for traumatic brain injury: quantifying neurofilament light chain (NfL) using silicon photonic biosensors"
2023 Optica Foundation Challenge $100,000 early career research grant: "Silicon photonic biosensors for low-cost, portable, data-rich measurements of hormone biomarkers relevant to women’s health and the menopausal transition"
2022 UBC Centre for Blood Research Dr. Neil Mackenzie Memorial Mentorship Excellence Award
2022 Rosalind Franklin Society Award in Science (Best 2021 Paper in Applied Biosafety)
MicroTAS 2020 Best Paper Award
UC President’s Lindau Nobel Laureate Meetings Fellow 2020 cohort
Johns Hopkins University ‘Rising Stars in Biomedical’ 2018 cohort
2017-2019 $45 000/yr NSERC Postdoctoral Fellowship
2017 Faculty nominee for Governor General’s Gold Medal Award (UBC)
2015 UBC Three Minute Thesis Competition University Wide Third Place
SLAS2015 Conference Best Student Poster Award
2014-2016 $30 000 Canadian Breast Cancer Foundation Doctoral Fellowship
2011-2014 $35 000/yr NSERC Alexander Graham Bell Canada Graduate Scholarship, PhD Level
2011-2014 $30 000/yr UBC Killam Doctoral Fellowship (accepted in name only)
2010-2011 $17 500 NSERC Alexander Graham Bell Canada Graduate Scholarship, Masters Level
$24 000 Simon Fraser University 5 year Dr Gordon Shrum Entrance Scholarship
Resume
>10 years of interdisciplinary research experience across 3 institutions, spanning silicon photonics, microfluidic design, sensor characterization, immunoassay development, and micro- and nanofabrication.
Education & Training
Postdoctoral Fellow
2017-2020: Postdoctoral Fellow, Herr Lab, Bioengineering and the California Institute for Quantitative Biosciences (QB3)
- Canada Natural Sciences and Engineering Research Council (NSERC) Postdoctoral Fellow
- Led a small team to introduce a new class of measurement tool for single-cell protein quantification
- Contributed to N95DECON’s scientific effort to help inform decision-making about N95 respirator decontamination
- Selected for the Johns Hopkins University 2018 cohort of ‘Rising Stars in Biomedical’
- Co-instructed at Cold Spring Harbor Laboratory’s 2019 Single Cell Analysis course
Ph.D. Student
2010-2016: Ph.D., Electrical and Computer Engineering
- NSERC CGS Scholar, Killam Scholar, Facultyof Applied Science nominee for Governor General’s Gold Medal
- 4.29/4.33 course GPA, 5 first-authored and 8 co-authored peer-reviewed journal publications
Undergraduate Student
2005-2010: B.A.Sc., Hons., Engineering Physics
- First Class Honours, Dean’s and President’s Honour Rolls, Honours Thesis Passed with Distinction.
- 3.96/4.33 Cumulative GPA, 4.14/4.33 Upper Division GPA, Dr. Gordon Shrum full five-year scholarship recipient.
Interests
- Addressing historically neglected needs for measurements, in areas like women's and menopausal health, using state-of-the art tools built upon solid understanding of driving fundamental mechanisms.
- Introducing robust, quantitative measurement workflows, leveraging interdisciplinary research, silicon photonics integration and system miniaturization, novel devices, multidimensional image processing, and data science.
- Quantifying dynamic cell state via phenotypic monitoring and precise molecular measurements.
- Design, optimization, and microfluidic integration of optical biosensors and chemical sensors.
- Harnessing small size scales and novel materials to recreate controlled environments to study cell and tissue behaviour.
Publications
- Highlights: 56 total refereed publications, including 29 journal publications, and >2100 citations.
- For a full list of publications, please see my Google Scholar.